Application Note
MaxCyte® Enabled Development of Allogeneic CAR T Cells as a Potential Therapy for Acute Myeloid Leukemia
Aim
In this study, researchers from Clinica Universidad de Navarra and Cima Universidad de Navarra used a novel, non-viral engineering approach to create allogeneic CD33-CAR-T cells (CD33-AlloCART) as a potential bridging treatment for rrAML2. The MaxCyte GTxTM simultaneously delivered two CRISPR RNPs, Sleeping Beauty 100X transposase (SB100X) mRNA and CD33-CAR transposon minicircle DNA, to generate functional CD33-AlloCART cells with a desirable phenotype and cytotoxic activity following small-scale and clinically significant medium-scale electroporation.
Workflow overview
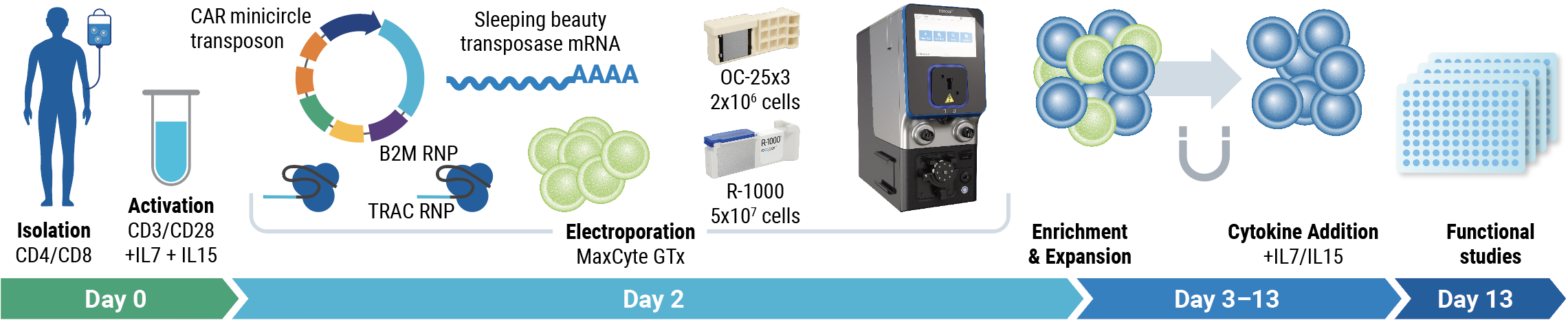
Background
Acute myeloid leukemia (AML) is an aggressive form of blood cancer that is primarily treated with chemotherapy. While many patients achieve complete remission, a significant number experience relapse or are diagnosed with refractory disease. The prognosis for these rrAML patients is particularly poor, with hematopoietic stem cell transplantation (HSCT) as the best treatment option. Although critical to successful HSCT, achieving remission of rrAML is challenging, and many patients are unable to benefit from this treatment option. CAR T therapy could be used to eliminate drug-resistant leukemic cells, enabling more rrAML patients to progress to HSCT.
Several autologous CAR T therapies have been approved to treat various relapsed or refractory blood cancers, and many new CAR T therapies are showing promising results in clinical trials. However, early attempts to develop autologous CAR T therapies to treat rrAML have had limited success. Possible causes include the effects of initial rounds of aggressive chemotherapy and the exhausted phenotype of rrAML patient T cells1.
In the decades since the first CAR T cells were created using viral vectors, the genetic engineering toolbox has expanded, enabling the development of safer, more cost-effective, and increasingly complex therapies. These advances may enable the development of allogeneic CAR T therapies using modified healthy donor T cells to produce “off-the-shelf” therapies, providing faster treatment with a reduced risk of GvHD and immune rejection.
Method
- Harvest: T cells were harvested from healthy donors
- Activation: CD4+/CD8+ T cells were cultured in the presence of IL-7 and IL-15, and activated with CD3/CD28
- Electroporation: The MaxCyte GTx simultaneously delivered CAR transposon minicircle DNA, SB100X mRNA, B2M RNP, and TRAC RNP
- Enrichment and Expansion: Negative magnetic enrichment selected double knockout (HLA-1- and TCR-) cells. Enriched cells were expanded in media containing IL-7 and IL-15
- Characterization: CD33-AlloCART cells were immunophenotyped, and their functionality was determined by cytotoxicity assays and cytokine production
AlloCART engineering strategy
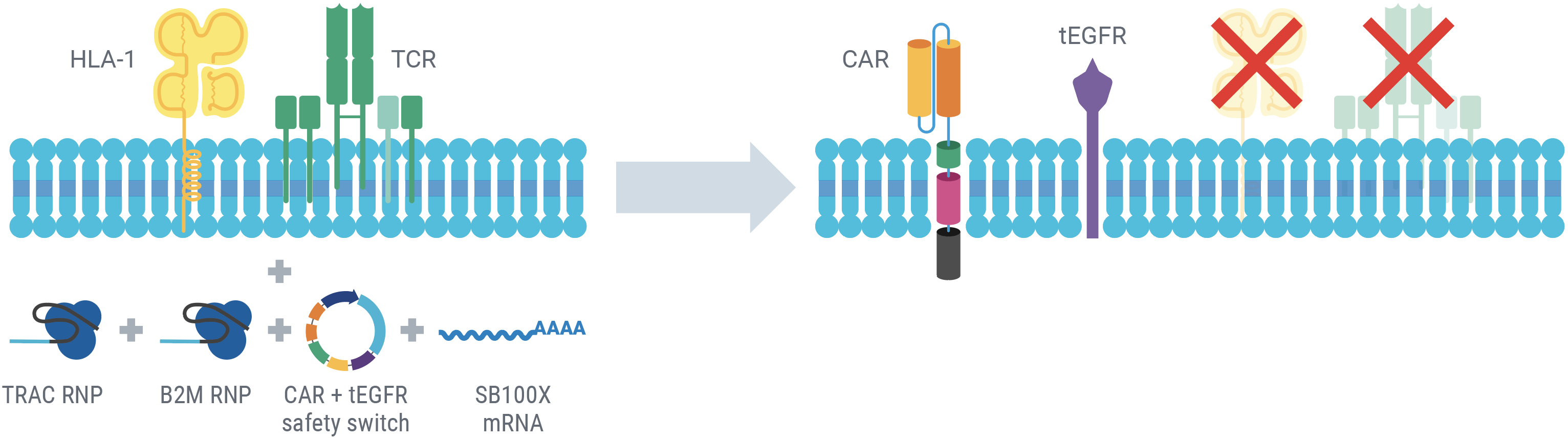
The simultaneous delivery of CAR transposon minicircle, SB100X mRNA, and TRAC and B2M RNPs enables a one-step engineering approach to knockout HLA-1 and TCR and knock in a CD33 CAR and a truncated human EGFR safety switch (tEGFR). Following electroporation, the persistence of transposase mRNA and CAR transposon minicircle DNA is transient, enhancing AlloCART safety3. The tEGFR safety switch enables the use of CD33 as a target antigen without risking potentially fatal myeloablation due to prolonged on-target, off-tumor cytotoxicity3. Here, engineered T cells with HLA-1 and TCR complex knockout and expressing CD33 CAR are referred to as CD33-AlloCART cells.
Results
Generation of CD33-AlloCART cells.
MaxCyte electroporation enabled the efficient one-step delivery of CD33-CAR transposon minicircle, SB100X mRNA, and CRISPR RNPs, yielding an average of 32.4% of CD33-CAR positive cells (Figure 1A). More than 69% of edited T cells were negative for both HLA-1 and TCR complexes (Figure 1B). Enrichment by negative selection yielded an almost pure population of double knockout allogeneic T cells (>98%) without reducing the proportion of cells expressing the CD33-CAR (Figure 1B, 1C).
A
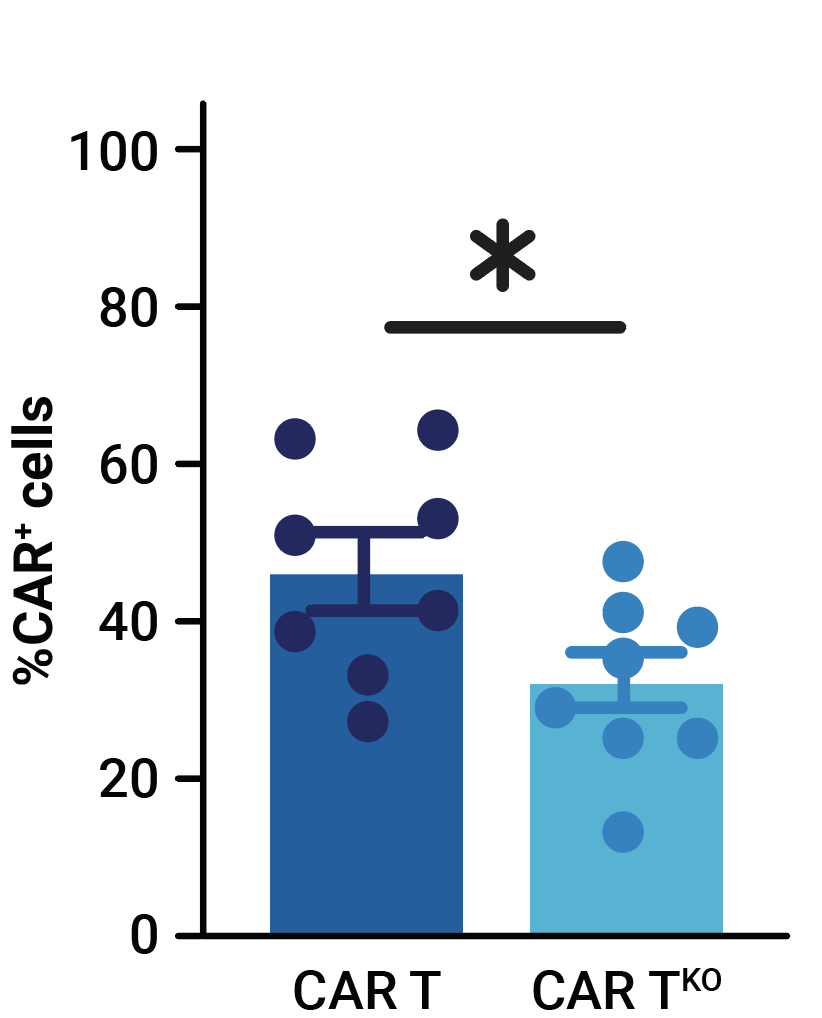
B
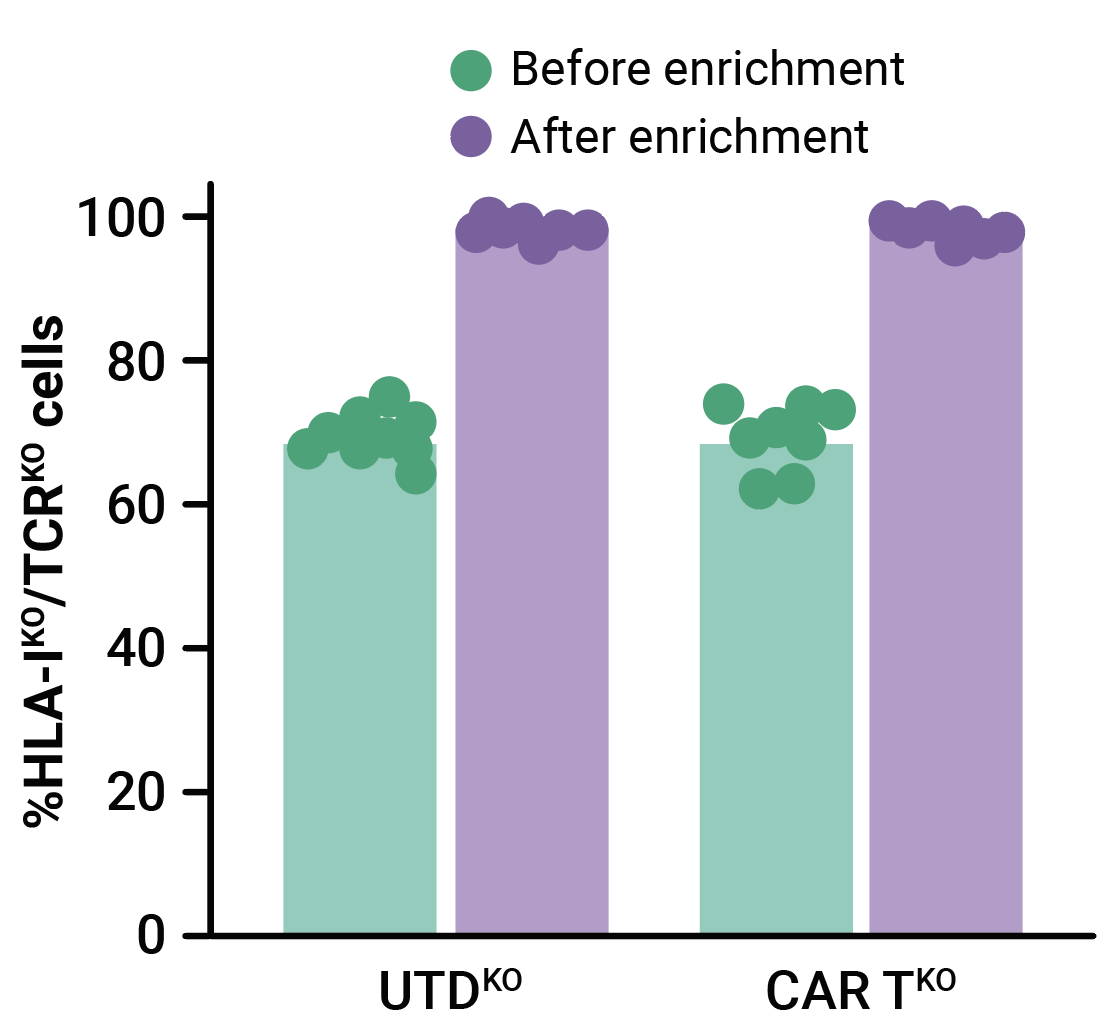
C
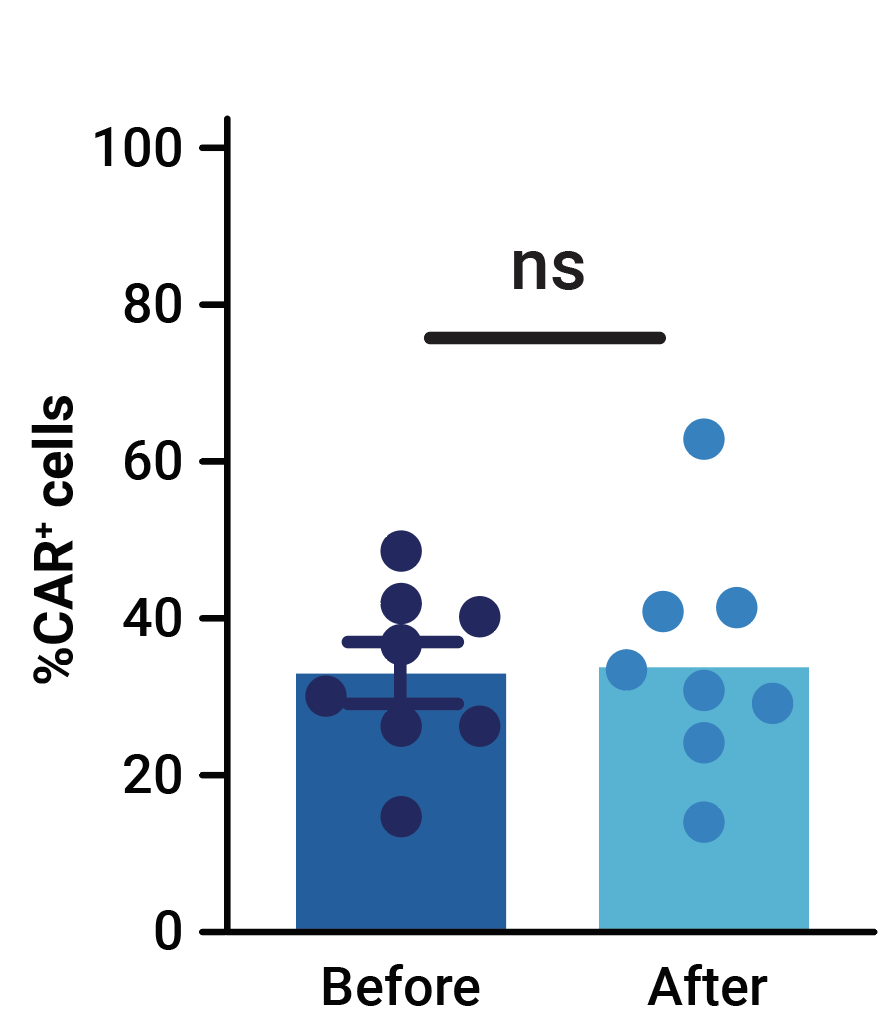
Figure 1. Phenotypic characterization of CD33-AlloCART cells generated from healthy donors (n=8). A) Percentage of cells expressing CD33-CAR following transposon/transposase delivery with or without CRISPR knockout. B) Percentage of HLA-1 and TCR complex double knockout in T cells with (CAR TKO) or without (UTDKO) CD33-CAR transfection before and after enrichment by negative selection. C) Percentage of CD33-CAR expressing cells before and after enrichment for double knockout cells. The mean ± SEM for each group is depicted. Kruskal-Wallis test with Dunn’s multiple comparisons test (A,C). ns = not significant; *p<0.05; ***p<0.001.
Characterization of CD33-AlloCART cells.
Compared with control cells, the proliferative capacity of CD33-CAR T cells, although slightly reduced, was not significantly impacted by HLA-1 and TCR complex knockout (Figure 2A). Phenotypic characterization of resting CD33-CAR T cells by flow cytometry confirmed that HLA-1 and TCR complex knockout did not alter the cell phenotype (Figure 2B). In vitro cytotoxicity towards CD33-expressing MOLM-13 AML cells (Figure 2C) and anti-tumor activity in an AML xenograft mouse model (Figure 2D) is consistent between CD33-CAR T cells (CAR T) or double knockout CD33-AlloCART cells (CAR TKO).
A
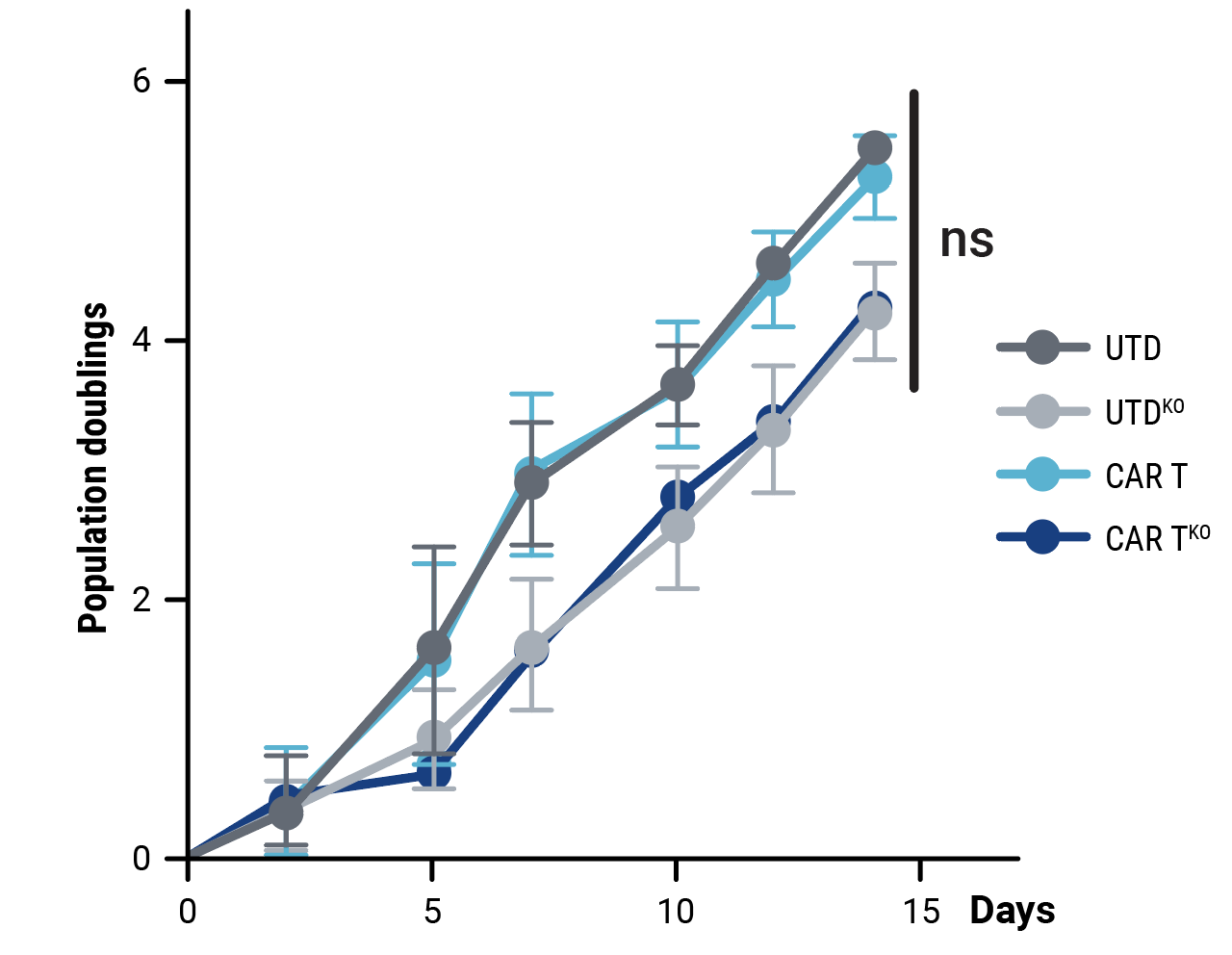
B
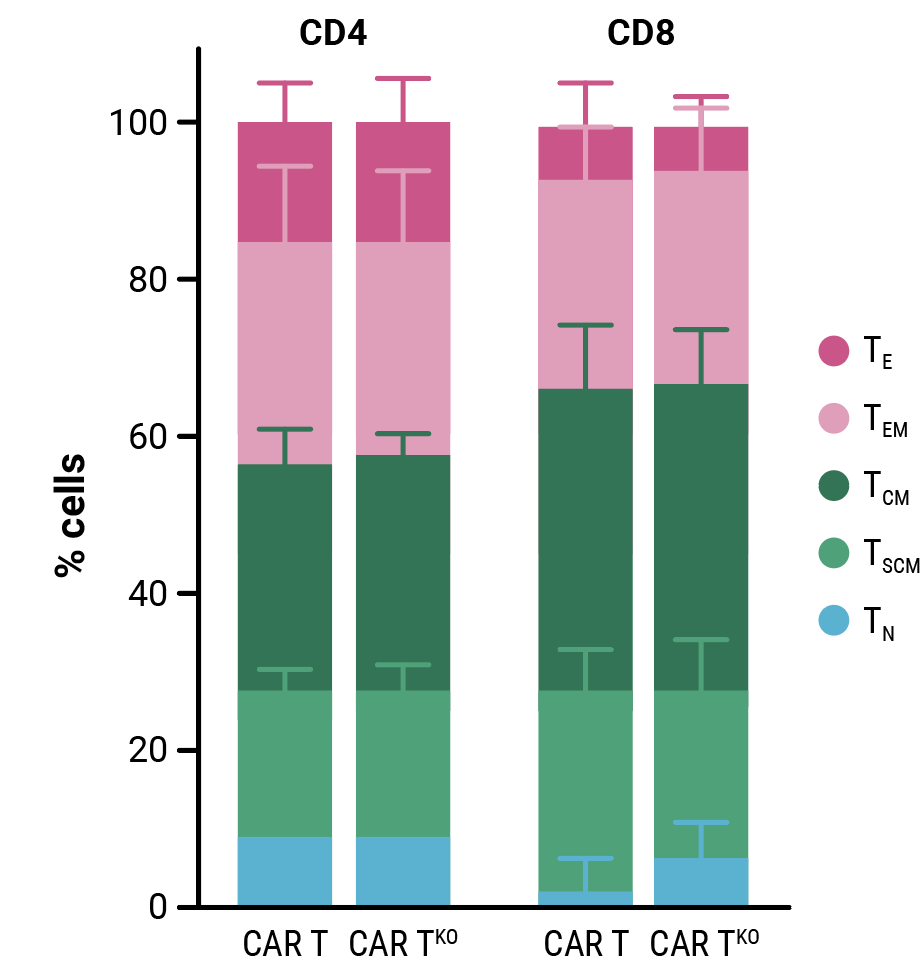
C
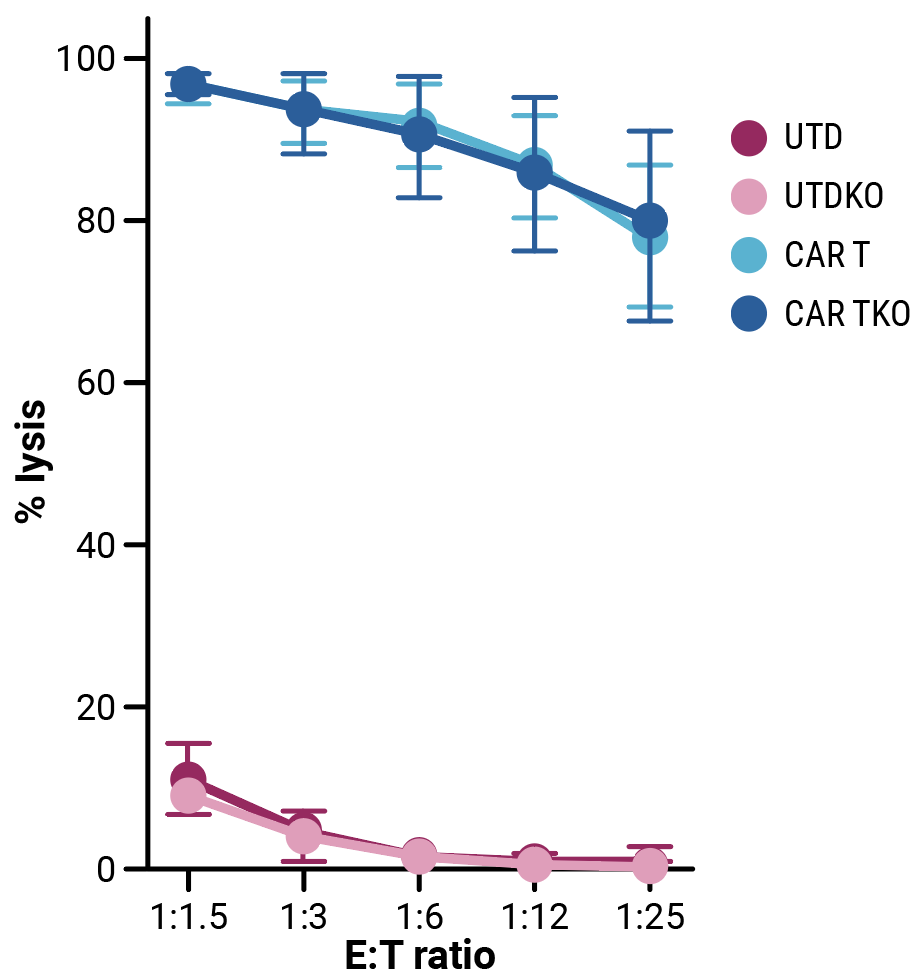
D
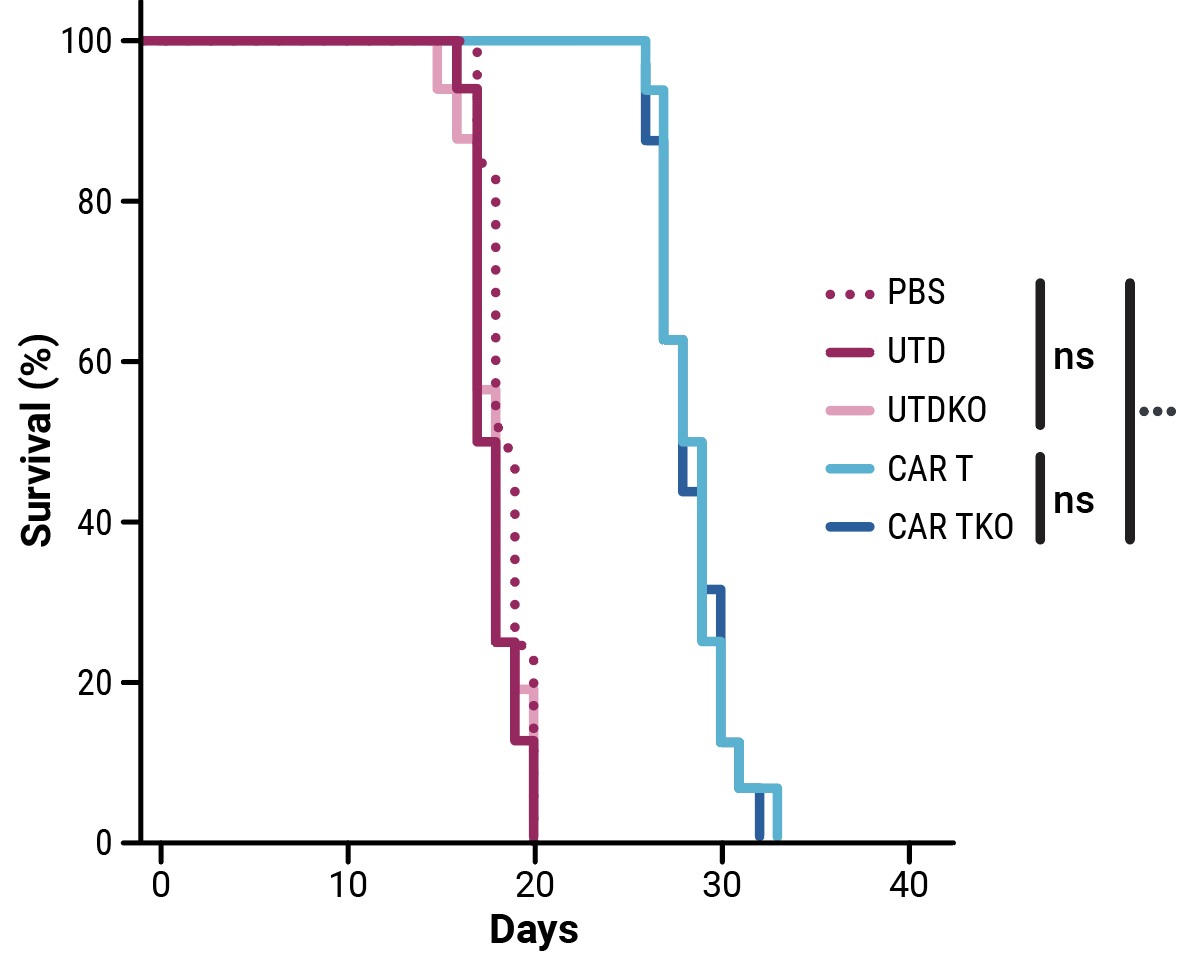
Figure 2. Functional characterization of CD33-AlloCART cells generated from healthy donors (n=8). A) Proliferation of CD33-CAR T (CAR T) and CD33-AlloCART (CAR TKO) cells during production. Untransfected (UTD) and HLA-1/TCR knockout (UTDKO) cells were used as controls. B) Analysis of the phenotype of resting CD33-CAR T (CAR T) and CD33-AlloCART (CAR TKO) cells divided by CD4+ and CD8+ T cells. TN, naïve; TSCM, stem central memory; TCM, central memory; TEM, effector memory; TE, effector. C) Specific lytic activity of CD33-CAR T (CAR T) and CD33-AlloCART (CAR TKO) cells against CD33+ MOLM-13 AML cells (average of three technical replicates). UTD and UTDKO cells were used as controls. D) Survival of mice treated with CD33-CAR T (CAR T) and CD33-AlloCART (CAR TKO) cells. Control animals were untreated (PBS) or treated with UTD or UTDKO cells. The mean ± SEM for each group is depicted. 2-way ANOVA with Tukey’s multiple comparisons test (A, C) and Logrank test (D). ns = not significant; *p<0.05; ***p<0.001.
CD33-AlloCART production can be seamlessly scaled up without altering phenotype or function.
To assess the scalability of MaxCyte electroporation and this novel one-step engineering strategy, CD33-AlloCART cells generated by either small-scale (2x106 cells) or clinically relevant medium-scale (5x107 cells) production were characterized. No difference was seen in the efficiency of CRISPR knockout (Figure 3A). Following enrichment by negative selection, there was no significant difference in the proportion of cells expressing the CD33 CAR (Figure 3B) or in the proliferative capacity of CD33-AlloCART cells (Figure 3C). Functional analysis showed no significant difference in the cytotoxic activity (Figure 3D) or cytokine production (Figure 3E) of CD33-AlloCART cells produced at either scale. Finally, MaxCyte electroporation of 5x107 donor T cells enabled the generation of over 3x108 purified CD33-AlloCART cells (Figure 3F).
A
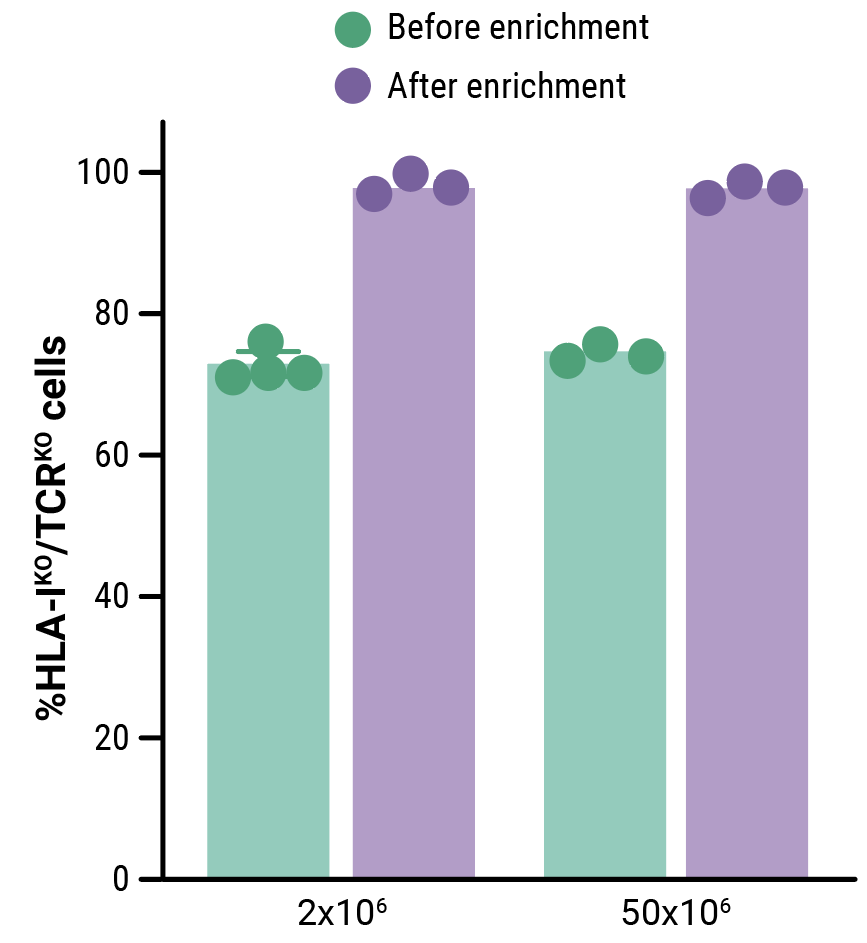
B
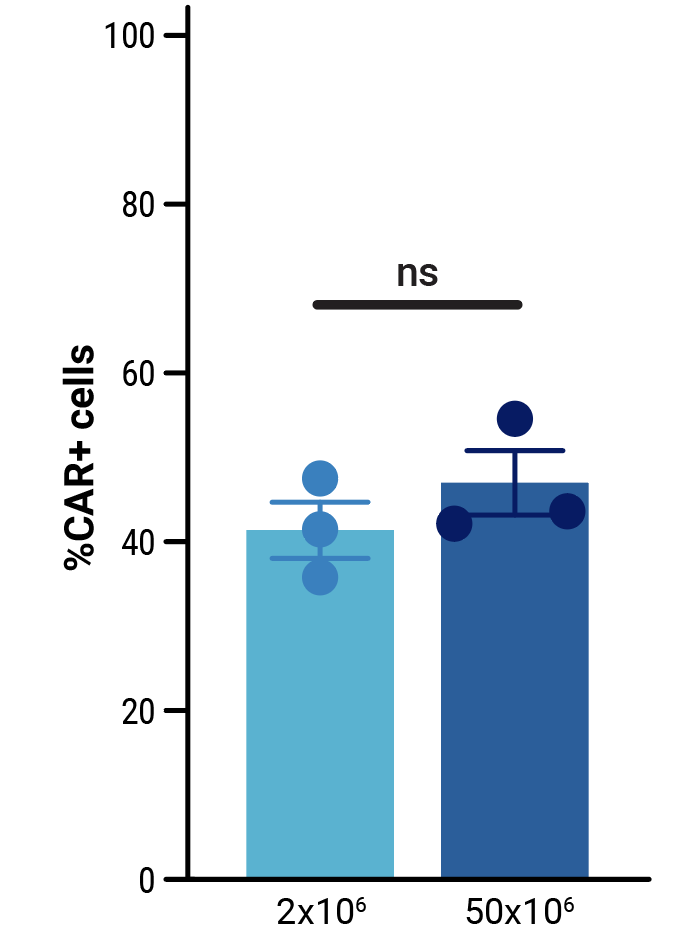
C
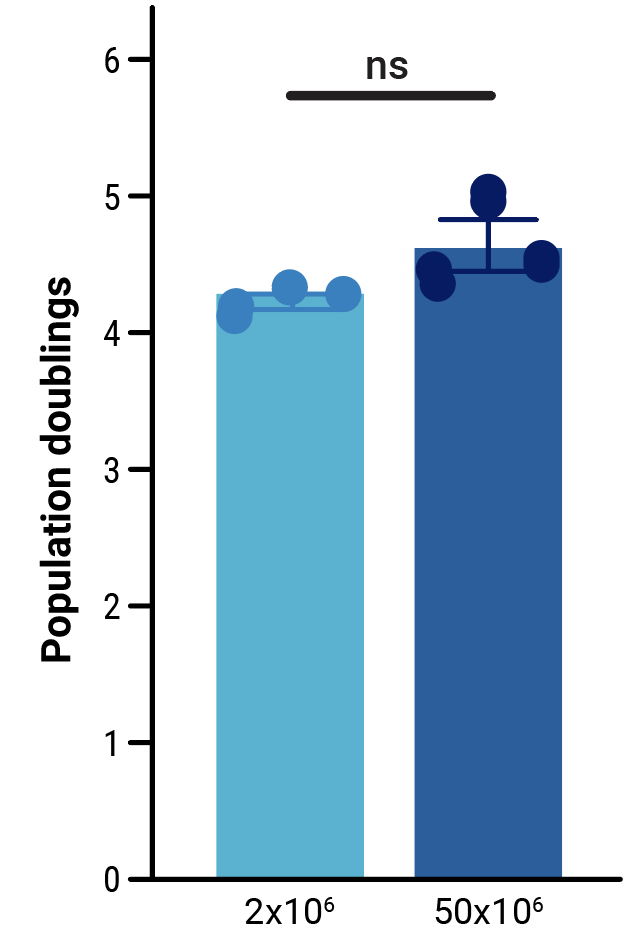
D
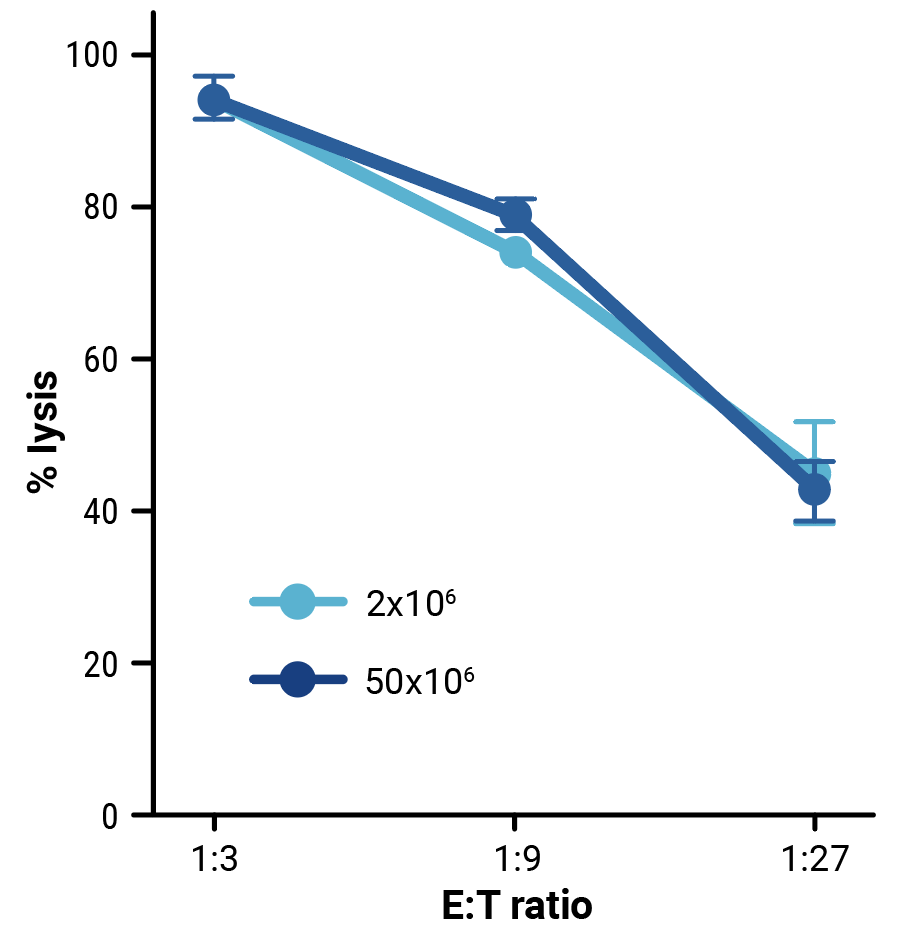
E
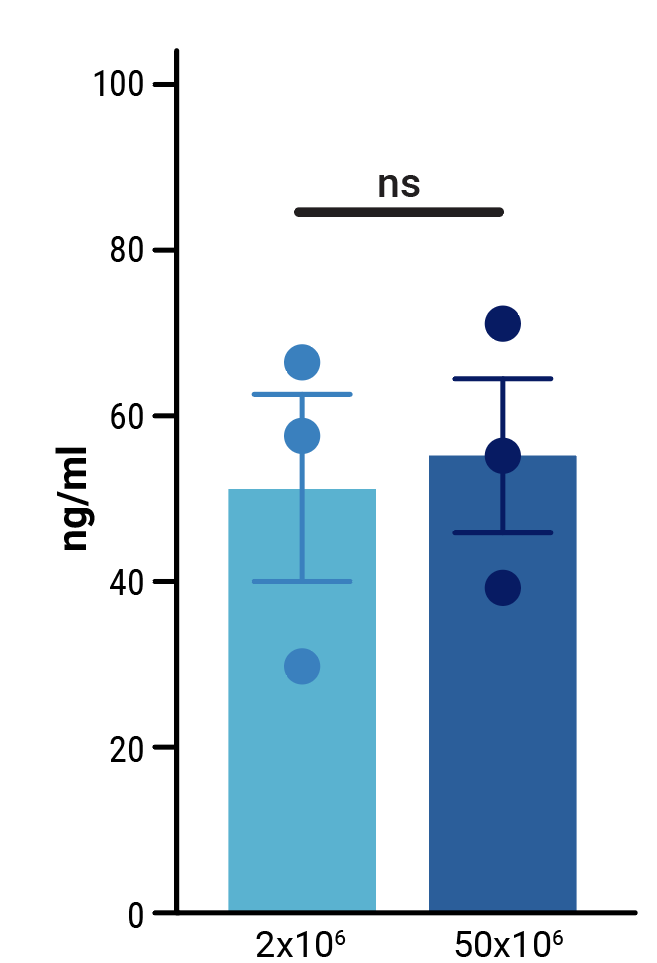
F
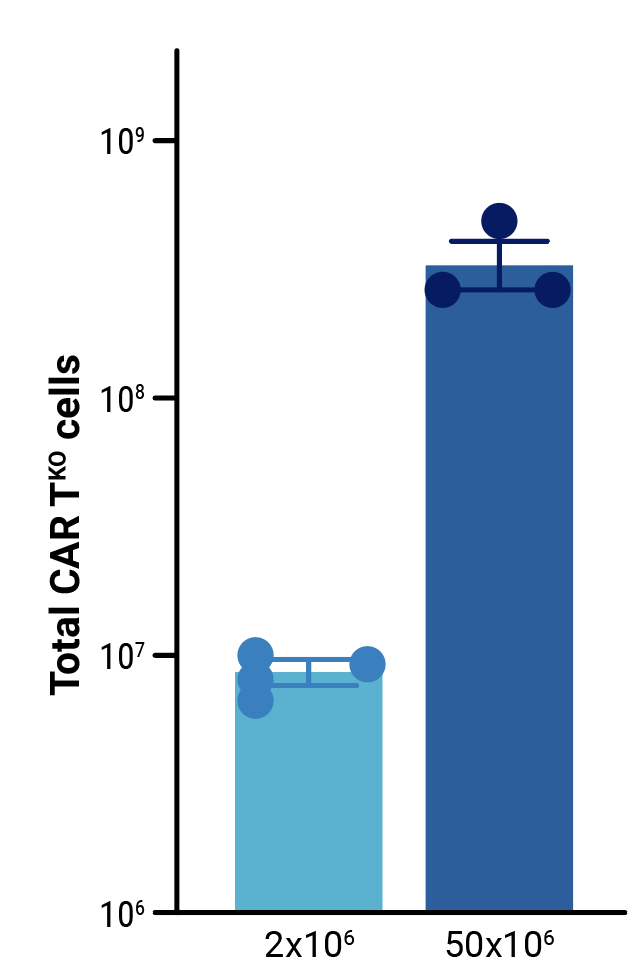
Figure 3: Characterization of CD33-AlloCART produced by small or medium-scale electroporation. A) Percentage of double knockout cells before and after selection (n=3 independent productions). B) Percentage of cells expressing CD33-CAR after selection for HLA-1 and TCR double knockout (n=3 independent productions). C) Proliferation of CD33-AlloCART cells during production (n=3 independent productions). D) Specific lytic activity of CD33-AlloCART cells against CD33+ MOLM-13 AML cells (average of three technical replicates) from three independent productions. E) ELISA quantification of the average IFN-γ level in supernatants from three technical replicate cytotoxicity assays (ratio 1:3) for each CD33-AlloCART cell production (n=3). F) Quantification of CD33-AlloCART cells obtained after enrichment and expansion (n=3 independent productions). Statistical analyses were done using the Mann Whitney test (B, C, E) or 2-way ANOVA with Tukey’s multiple comparisons test (D). ns = not significant.
Conclusion
This study describes the development and validation of a method for one-step Sleeping Beauty driven CAR knockin with double CRISPR knockout to create healthy, functional CD33-AlloCART cells.
The ability of MaxCyte electroporation to simultaneously co-deliver minicircle DNA, transposase mRNA and CRISPR RNPs to primary human T cells without altering cell phenotype and fitness was critical to the success of this approach.
MaxCyte’s clinically proven ExPERT GTx system was used to produce CD33-AlloCART at a clinically relevant scale without the time limitations or high manufacturing costs associated with viral vector-based therapies. CD33-AlloCART cells have great potential as a treatment option for rrAML patients before HSCT.
Related Resources
References
- Naik S, Velasquez MP, Gottschalk S. (2024) Chimeric antigen receptor T-cell therapy in childhood acute myeloid leukemia: how far are we from a clinical application?. Haematologica 109(6):1656-1667; https://doi.org/10.3324/haematol.2023.283817.
- Calviño C, Ceballos C, Alfonso A, Jauregui P, Calleja-Cervantes ME, San Martin-Uriz P, Rodriguez-Marquez P, Martin-Mallo A, Iglesias E, Abizanda G, Rodriguez-Diaz S, Martinez-Turrillas R, Illarramendi J, Viguria MC, Redondo M, Rifon J, Villar S, Lasarte JJ, Inoges S, Lopez-Diaz de Cerio A, Hernaez M, Prosper F and Rodriguez-Madoz JR (2023) Optimization of universal allogeneic CAR-T cells combining CRISPR and transposon-based technologies for treatment of acute myeloid leukemia. Front. Immunol. 14:1270843. doi: 10.3389/fimmu.2023.1270843
- Data generated in collaboration with CIMA/Clinica Universidad de Navarra and CIEMAT. Adapted with permission.