Application Note
MaxCyte® Enables Multiplexed NK Cell Engineering
Experimental Design
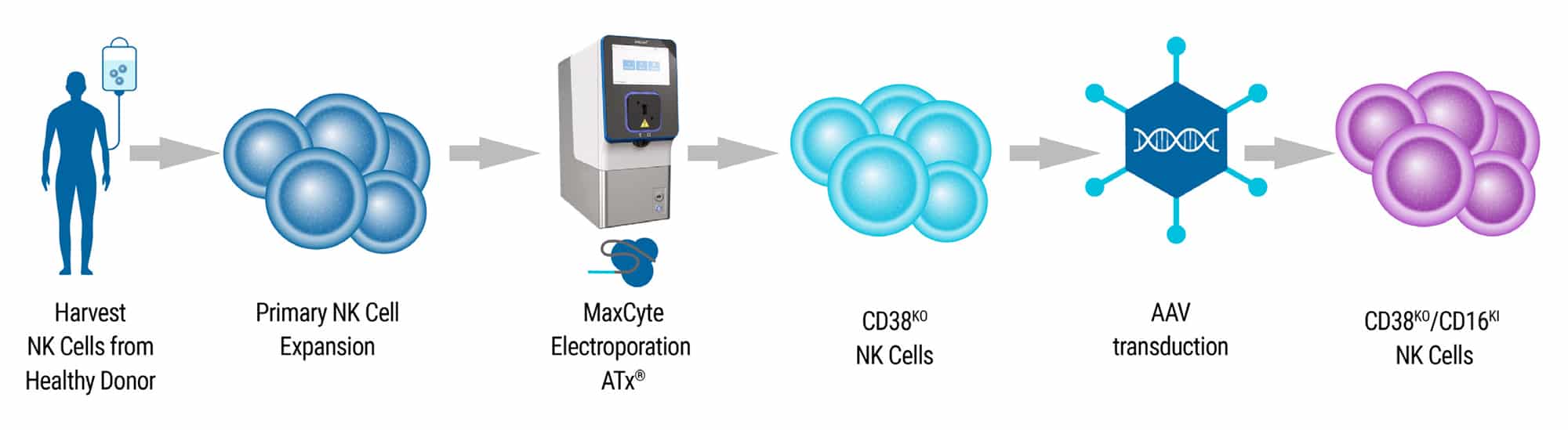
Background
As the immune system’s first responders, natural killer (NK) cells are essential for the rapid detection and elimination of cancer. Their ability to kill cancer cells without prior sensitization makes them an ideal immunotherapy candidate. NK cells induce an anti-tumor immune response known as antibody-dependent cellular cytotoxicity (ADCC) when activating receptors, such as CD16, bind to monoclonal antibodies (mAbs) on target cells. Combining adoptive NK cell infusions with mAbs is a promising strategy for treating cancer.
Daratumumab (DARA) is a mAb that binds to CD38 and has shown clinical efficacy in treating multiple myeloma, making it an attractive candidate to augment NK cell infusion. However, as circulating NK cells express high levels of CD38, DARA can cause ADCC-dependent NK fratricide, limiting the efficacy of this therapeutic combination1 . A strategy combining DARA with the adoptive transfer of CD38 knockout (CD38KO) NK cells has the potential to maximize ADCC and prevent DARA-induced fratricide, enhancing NK cell persistence. To further augment their efficacy, NK cells can be engineered to express a high-affinity CD16 (CD16-158V) receptor, which has been shown to boost ADCC, resulting in improved clinical outcomes2.
Here, we demonstrate how MaxCyte® enabled multiplexed genetic engineering in NK cells. Dual-edited NK cells improved DARA treatment and strengthened anti-tumor responses in vitro and in vivo. MaxCyte electroporation provided significant benefits including high efficiency, low toxicity and clinical scalability, empowering the rapid development of a potent cancer therapeutic.
Aim
This exciting study from the Childs lab used complementary genetic engineering strategies to generate NK cells with enhanced ADCC potency and resistance to fratricide in the presence of DARA. The experiments featured here demonstrate that MaxCyte electroporation had minimal effect on cell viability and function and is a safe platform to aid the development of cell-based cancer therapeutics.
Methods:
- Harvest NK cells were harvested from healthy donor peripheral blood buffy coat.
- Expansion Isolated NK cells were expanded ex vivo for one week. NK cells were cocultured with irradiated Epstein–Barr virus transformed lymphoblastoid feeder cells and IL-2.
- Electroporation The MaxCyte ATx® and NK-04 electroporation protocol were used to deliver precomplexed Cas9 and CD38-targeting gRNA to expanded NK cells. Knockin (KI) of CD16-158V was accomplished with two separate approaches: electroporation or AAV transduction.
- Transient CD16 Expression The ATx® was used to deliver CD16-158V mRNA to CD38KO NK cells 7 days after the first electroporation resulting in transient dual-edited NK cells.
- Stable CD16 KI Recombinant adeno-associated virus (rAAV6) containing an HDR template encoding the CD16-158V gene with an N-terminal FLAG-tag was added to CD38KO NK cells 30 minutes following initial electroporation. Transduction produced stable CD16-158V transgene integration in the CD38 locus.
- Characterization Engineered cells were isolated and characterized by PCR and flow cytometric analysis. The functionality of isolated NK cells was determined by an in vitro killing assay and in murine models of multiple myeloma.
Results
Highly efficient gene knockout
MaxCyte enabled efficient knockout of CD38 in 92% of the expanded NK cells (Fig 1A). Electroporation was gentle on cells as viability remained high several days following transfection (Fig 1B).
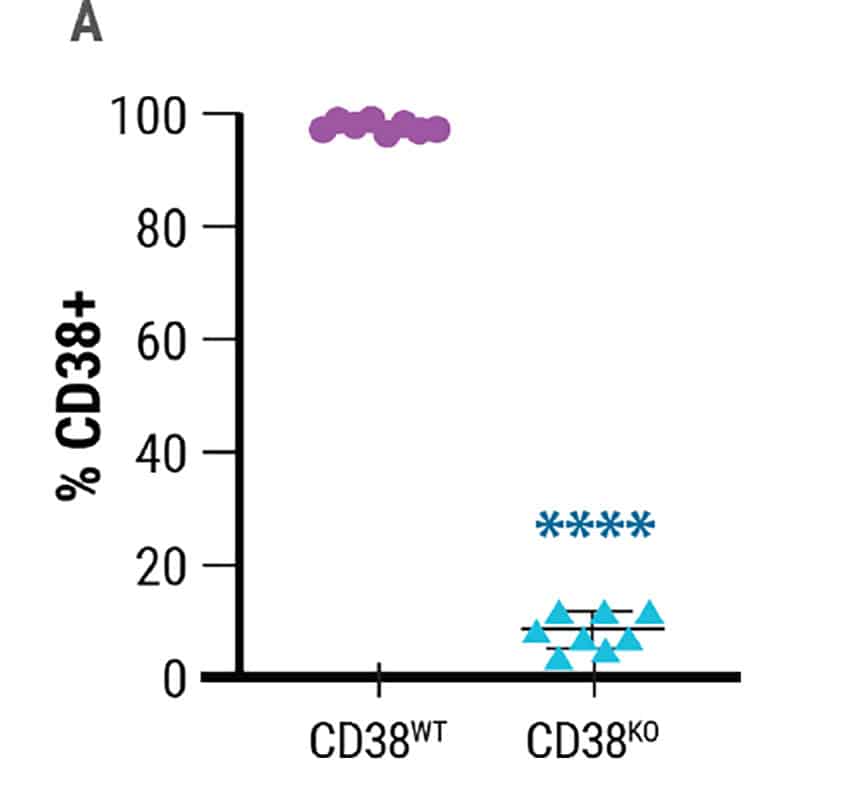
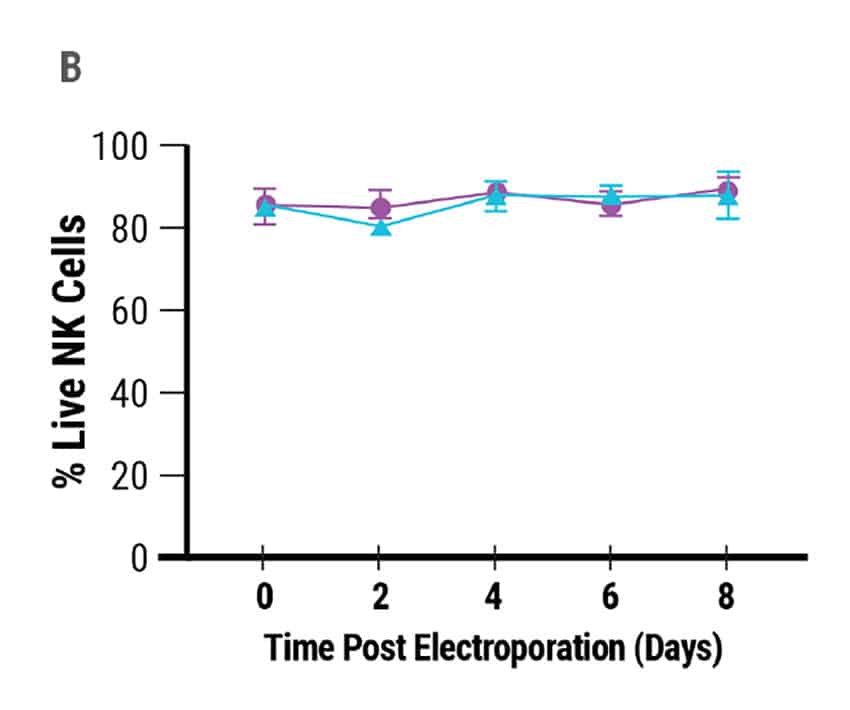
Figure 1. High-efficiency gene editing A) Relative expression of CD38 in wild-type (CD38WT) and CD38KO NK cells. B) Annexin staining and flow cytometry were used to determine NK cell viability 8 days post electroporation.
Characterization of CD38KO NK cells
Genetic engineering did not alter the proliferative or functional characteristics of NK cells. Unedited and CD38KO NK cells had similar rates of ex vivo expansion (Fig 2A) and the expression of a panel of NK surface receptors was not significantly altered post electroporation (Fig 2B). Degranulation was determined by measuring the expression of lysosomal-associated membrane protein-1(CD107a) on NK cells in the presence of their target cells and was similar in CD38KO and CD38WT cells (Fig 2 C). Additionally, NK cellular cytotoxicity was comparable between unedited and engineered NK cells as determined through the production of intracellular IFNү (Fig 2D) and TNFα (Fig 2E) in the presence of target cells.
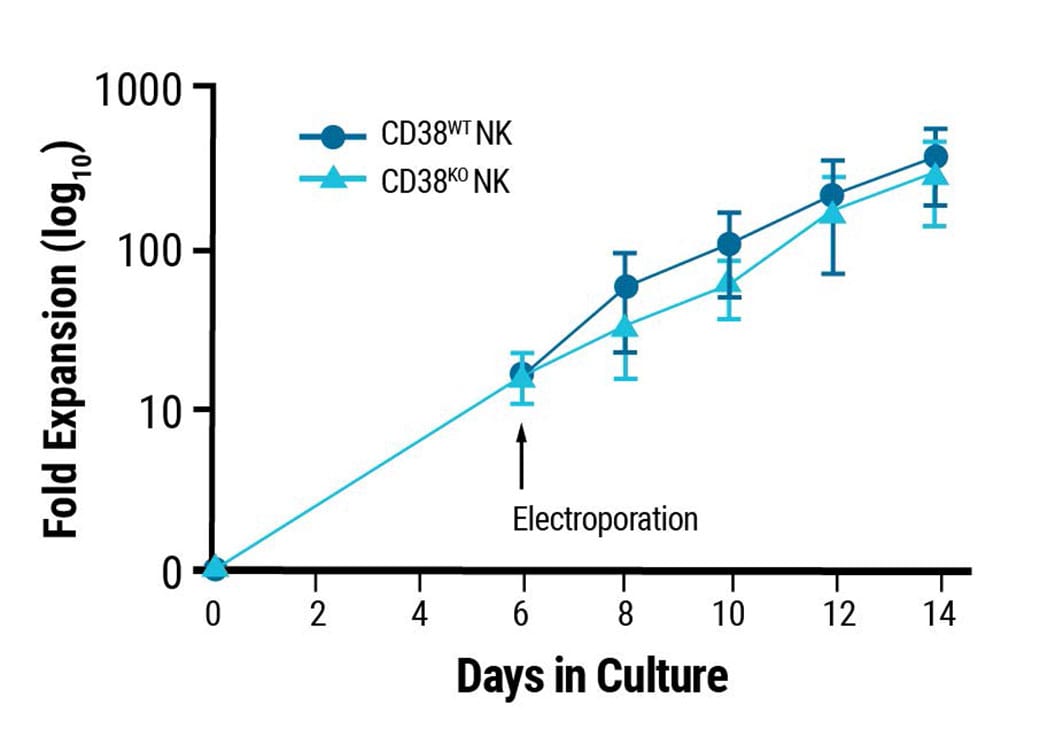
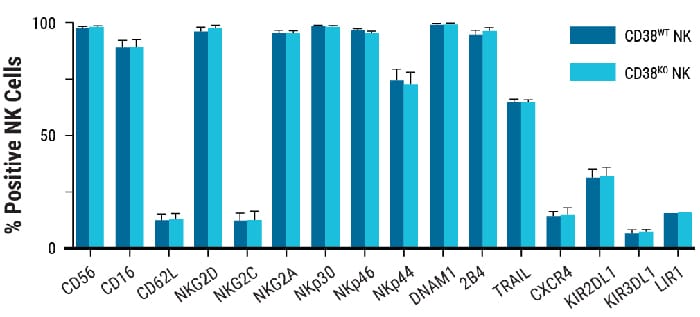
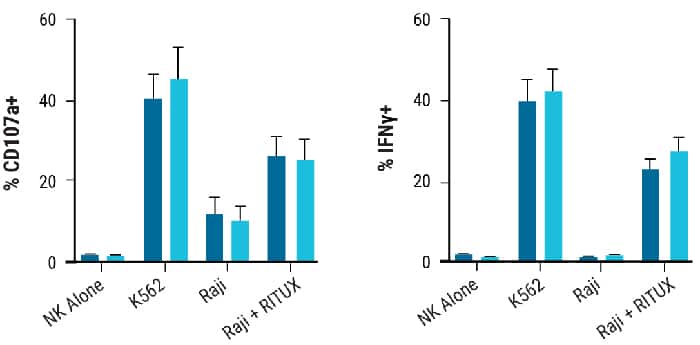
Figure 2. NK cell characterization post electroporation Proliferative and functional characteristics of NK cells remain unchanged post electroporation. A) CD38KO and CD38WT NK cell expansion 14 days post electroporation. B-E) Marker expression in CD38KO and CD38WT NK cells following coculture with target cells (K562 or Raji cells) was determined using flow cytometry. Raji cells treated with rituximab (RITUX) were used as a positive control. B) Surface marker expression in CD38KO and CD38WT NK cells. C) Degranulation was measured via CD107a expression. D-E) NK cytotoxicity was determined through intracellular INF-ү and TNF-α expression.
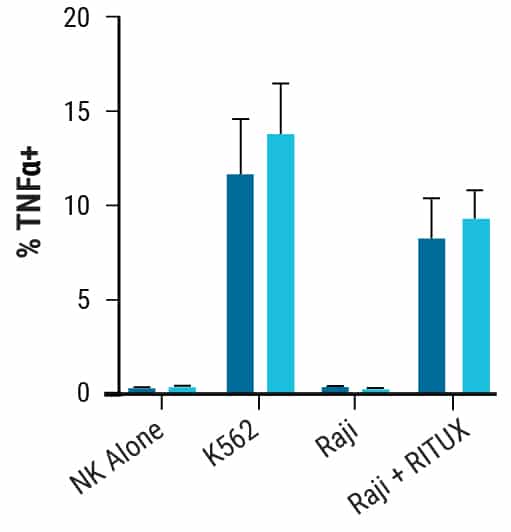
Arming CD38ᴷᴼ NK cells
To determine if the addition of CD16-158V could augment the potency of CD38KO NK cells, electroporation was used to deliver CD16-158V mRNA to engineered cells. CD38KO NK cells transfected with CD16-158V mRNA demonstrated superior cell lysis when cocultured with CD38+ NCI-H929 myeloma cells treated with DARA (Fig 3). MaxCyte electroporation was used to confirm that the efficacy of CD38KO NK cells could be further enhanced with the expression of CD16-158V.
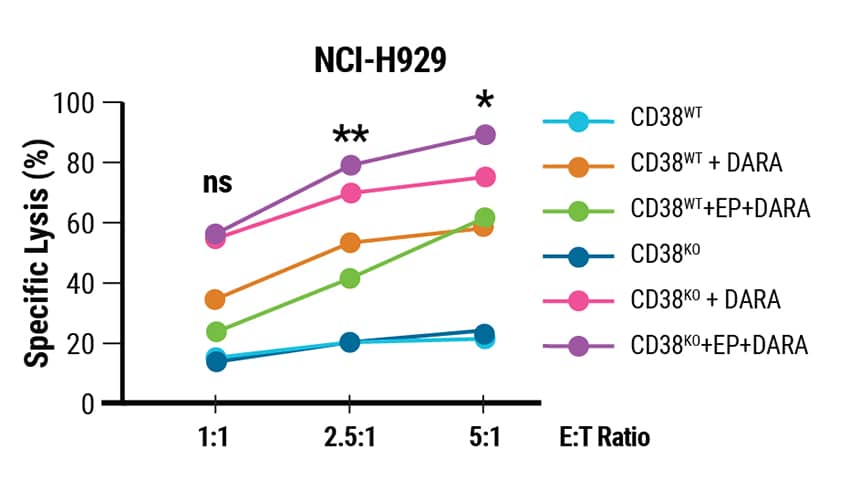
Figure 3. Enhanced functionality of CD38KO NK with CD16-158V expression Cell lysis of DARA-treated NCI-H929 cells in the presence of CD38KO NK cells with (CD38KO +EP) or without CD16-158V expression one day post electroporation at various effector-to-target (E:T) ratios.
Multiplexed Engineering
Stable multiplexed engineering was accomplished through the combination of electroporation and AAV transduction. CRISPR-Cas9 RNP electroporation was used to disrupt CD38 expression while a recombinant AAV delivered an HDR template encoding CD16-158V. Generation of double-edited CD38KO/CD16KI NK cells was determined by surface FLAG expression; 58% of CD38KO cells expressed CD16-158V (Fig 4A). Double-edited CD38KO/CD16KI NK cells in combination with DARA induced increased cell lysis in multiple myeloma target cells (Fig 4B) and showed the greatest reduction in tumor burden in MM1.S xenograft mice, demonstrating superior anti-tumor activity (Fig 4C). MaxCyte’s electroporation technology can be used with complementary genetic engineering tools, such as AAVs, to develop the next generation of NK cell therapeutics.
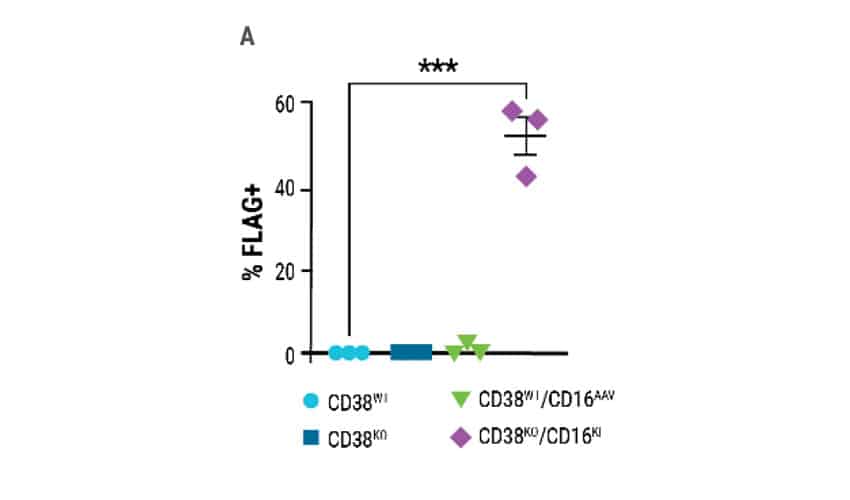
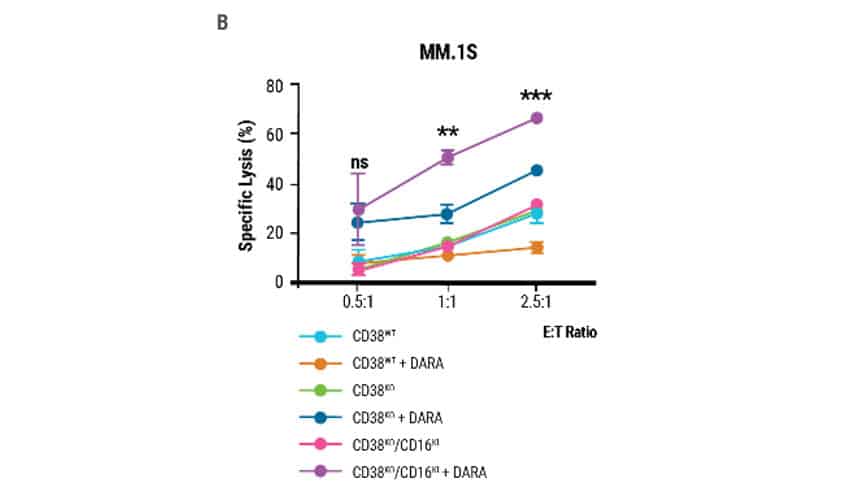
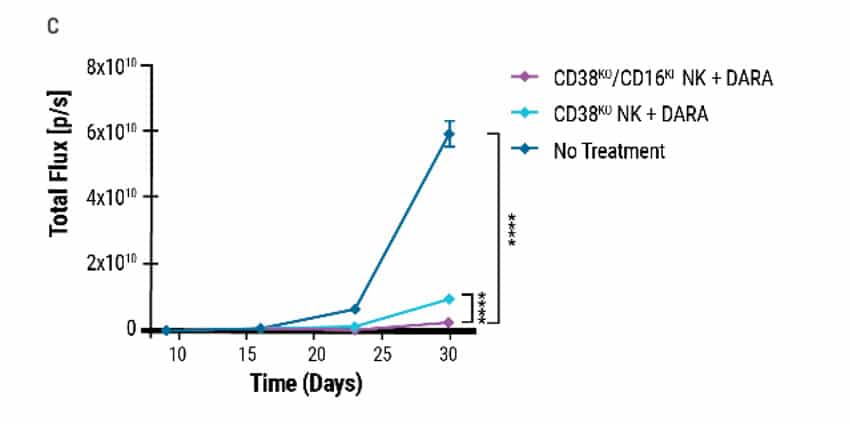
Figure 4. Double-edited NK cells have superior anti-tumor activity A) Percentage of FLAG-positive NK cells measured 7 days post CRISPR-Cas9 RNP electroporation and AAV transduction. B) Comparison of double-edited (CD38KO/CD16KI), single-edited (CD38KO) and unedited (CD38WT) NK cells’ ability to enhance DARA-mediated lysis in MM.1S target cells. C) Double-edited NK cells demonstrated higher anti-myeloma activity in xenograft mice. The MM.1S cells used to form the tumor were lentivirally transduced to express firefly luciferase. Bioluminescent quantification (photons per second or total flux) of MM.1S myeloma tumor growth was measured over 30 days.
Conclusion and Future Applications
Combining highly potent adoptive NK cell transfer with monoclonal antibody therapy is a promising cancer treatment strategy. MaxCyte’s electroporation technology paired with AAV transduction enabled the development of an easily adaptable workflow for multiplexed genetic engineering in NK cells. The high efficiency of CD38 knockout achieved with MaxCyte electroporation eliminated the need for additional enrichment steps for engineered cells. Additionally, the reduction of CD38 expression was stable over time and conferred resistance to DARA-induced NK fratricide. Electroporation did not compromise edited NK cells’ functional or proliferative capacity. MaxCyte electroporation was also used to arm CD38KO cells with the high-affinity CD16-158V, enhancing their cytotoxicity in the presence of DARA. The combination of electroporation and AAV transduction resulted in the efficient production of double-edited NK cells. CD38KO/CD16KI NK cells augmented DARA’s anti-tumor activity both in vitro and in vivo. MaxCyte enabled the development of a streamlined workflow for multiplexed engineering of NK cells that, when combined with DARA, represents an exciting combinatorial immunotherapeutic strategy perfectly positioned to advance into the clinic.
References
- Wang Y, Zhang Y, Hughes T, et al. Fratricide of NK Cells in Daratumumab Therapy for Multiple Myeloma Overcome by Ex Vivo-Expanded Autologous NK Cells. Clin Cancer Res. 2018;24(16):4006-4017. doi:10.1158/1078-0432.CCR17-3117.
- Clara JA, Levy ER, Reger R, Barisic S, Chen L, Cherkasova E, Chakraborty M, Allan DSJ, Childs R. High-affinity CD16 integration into a CRISPR/Cas9-edited CD38 locus augments CD38-directed anti-tumor activity of primary human natural killer cells. J Immunother Cancer. 2022 Feb;10(2):e003804. doi: 10.1136/jitc-2021-003804. PMID: 35135865; PMCID: PMC8830298.